Welcome to the Atomic and Quantum Optics research group at the School of Physics, University of Melbourne.
Here, we study how light interacts with all sorts of quantum systems and ways we can tailor this interaction for useful purposes.
We have two main research directions:
- Quantum sensing with nitrogen-vacancy centres in diamond: nanoscale quantum sensing with diamond defects
- Ultracold atoms, ions and electrons: using techniques from laser cooling and trapping to generate beams of cold electrons and ions
Now led by DECRA Fellow Dr Alex Wood, we have ongoing collaborations with both academic and industrial partners:
NV-diamond quantum sensing
Laser optimisation and frequency stabilisation
To find information about our group, our research interests and our publications, please check out the Menu bar in the top right-hand corner.
Our latest work on NV diamond geometric phases, in collaboration with the group of Prof Martin Plenio (Univ. Ulm, Germany) is detailed in a new preprint entitiled "Interplay between geometric and dynamic phases in a single spin system". In it, we look at what 'geometry' really means when a quantum system accumulates a phase in a cyclic evolution.
Our paper on detecting phase shifts induced by physical rotation of a single NV centre has been published in Physical Review Letters as an Editor's Suggestion, with an accompanying Focus article. This is the first time a quantum phase from classical rotation has been detected at the single-spin level, and these experiments demonstrate the fundamental connections between spin, physical rotation, and quantum phase. These results will be important for the development of nanoscale, diamond-based gyroscopes and other precision sensors where the rotational degree of freedom of a quantum system is not fixed, such as trapped nanoparticles containing spins.
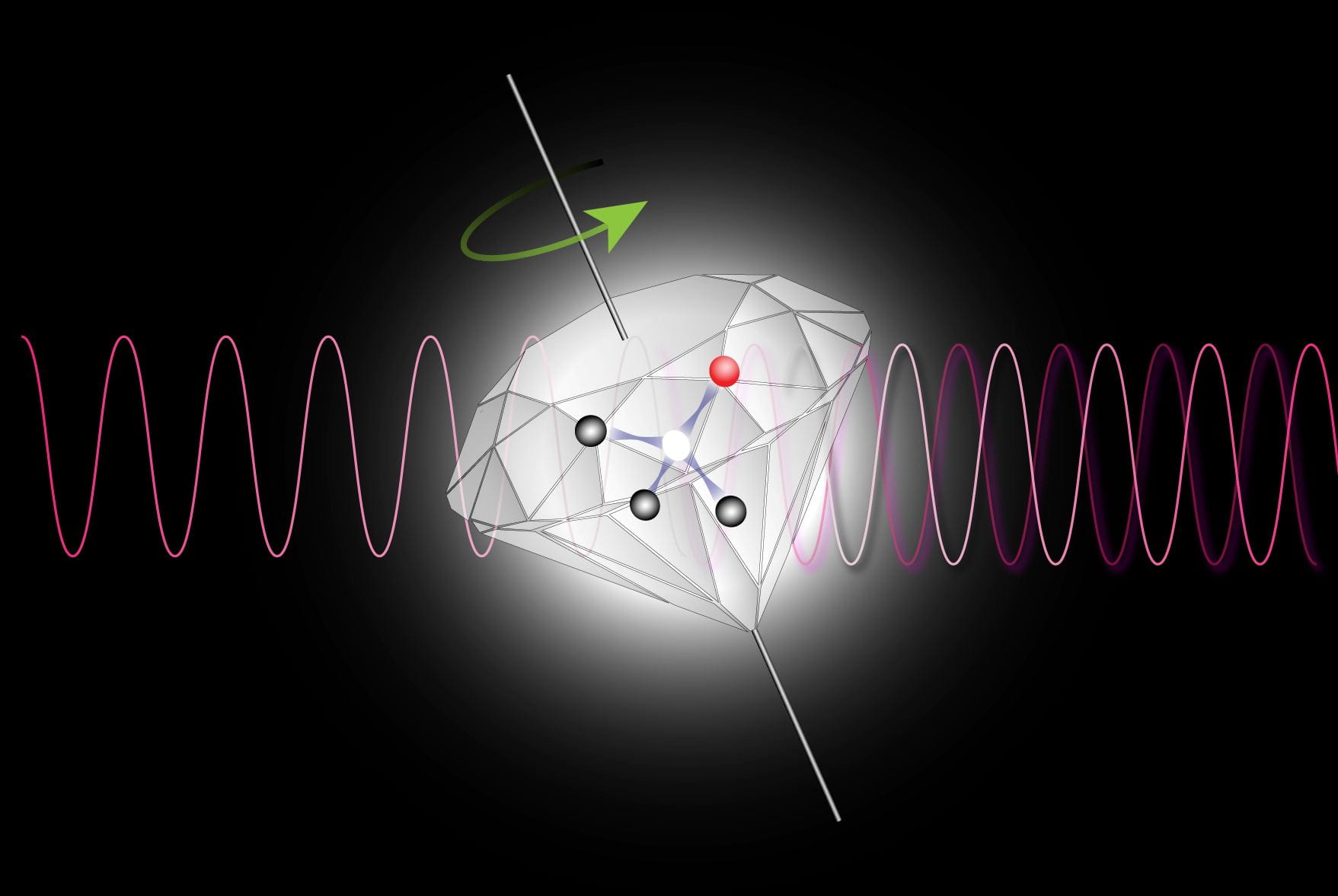
D. J. Thompson, D. Murphy, R. W. Speirs, R. M. W. van Bijnen, A. J. McCulloch, R. E. Scholten, B. M. Sparkes Physical Review Letters 117, 193202 (2016) [pdf]
Imaging with electron and ion beams is slow because the charges repel each other. Beams of electrons or ions diffuse because the particles repel each other via the Coulomb force, effectively reducing the focus and resolution for higher charge density beams. To image dynamic processes, single bunches of approximately 1 million electrons must be focused onto a sample that is only a few tens of micrometres across, to perform single-shot ultrafast coherent electron diffraction.
A source of electrons or ions based on photoionisation of cold atoms provides us with the ability to precisely shape the initial bunch by controlling the laser beam profiles used to ionise the atoms. For certain shapes, the Coulomb-driven expansion is linear, allowing us to reverse the expansion and more tightly focus the high-density beam. In this paper we present the first results demonstrating the reversal of the Coulomb explosion using three-dimensional shaping of the initial bunch, and show how certain distributions focus much better than others. These results have a significant impact for applications ranging from femtosecond chemistry to materials science and rational drug design.
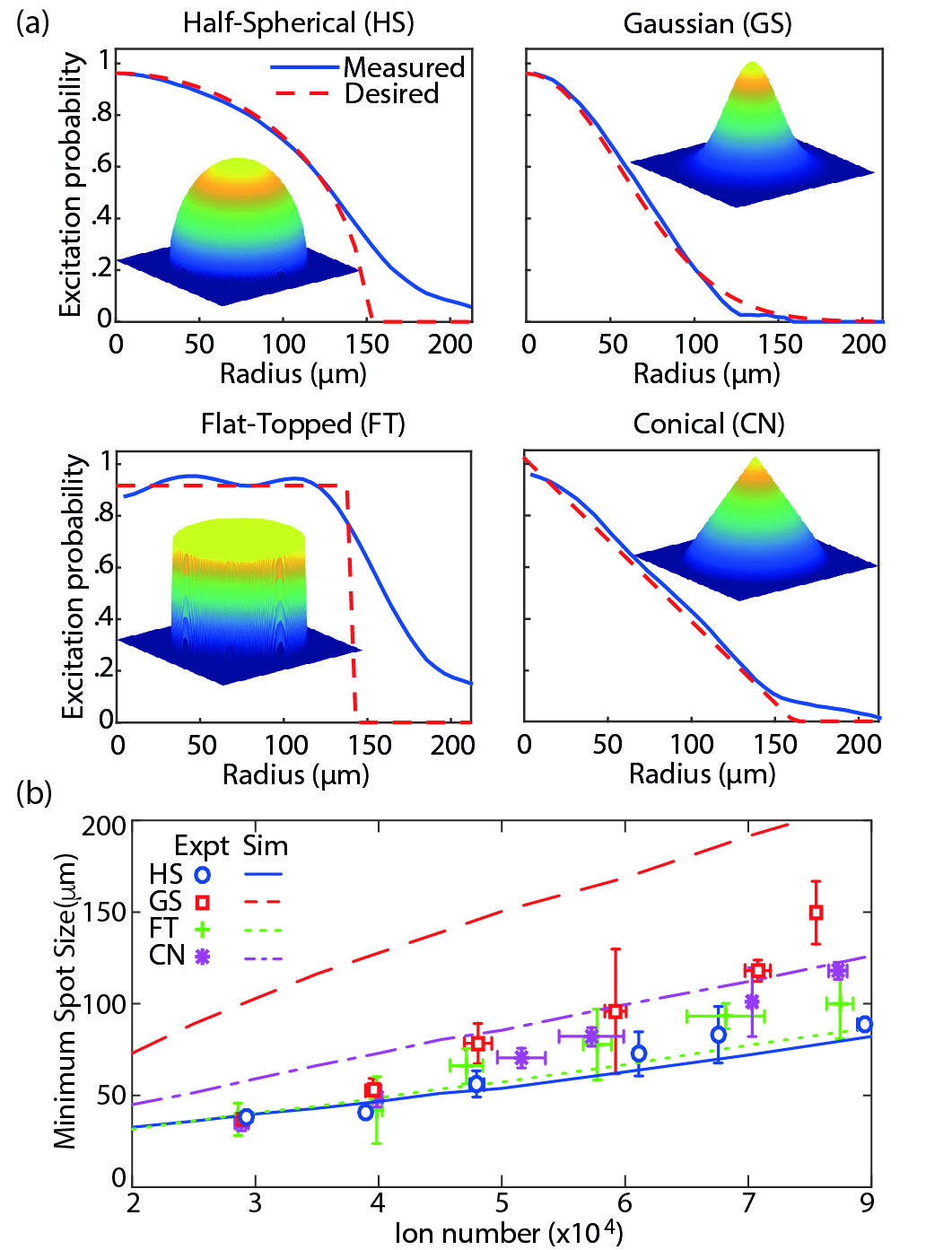
(a) Measured radially averaged excitation laser profiles (solid lines) and desired profiles (dashed lines), plotted as the relative excitation probability. These correspond to the transverse density profile of the electron and ion bunches created via photo-ionisation. Insets show desired transverse bunch density profiles as shaded false-colour renderings. (b) Measured minimum focused spot sizes for the different shaped distributions as a function of the number of ions present (points) and simulated focusability (lines).
The fabrication of ever-shrinking semi-conductor devices requires focused ion milling sources with smaller and smaller resolution. To achieve the sub-nanometre focusing potential of a cold atom electron and ion source (orders of magnitude smaller than thermal sources) will require the removal of any heating mechanisms, such as disorder-induced heating. One way of overcoming this effect is to place atoms in a structured array created with a 3D optical lattice. Experimentally filling 100% of lattice sites is, however, extremely challenging. Here we calculate the heating for fillings from 0 to 99%, showing that even imperfect filling can still lead to a large reduction in the negative effects of disorder-induced heating.
Reference: D. Murphy, B. M. Sparkes, Physical Review E 94, 021201(R) (2016) [pdf]
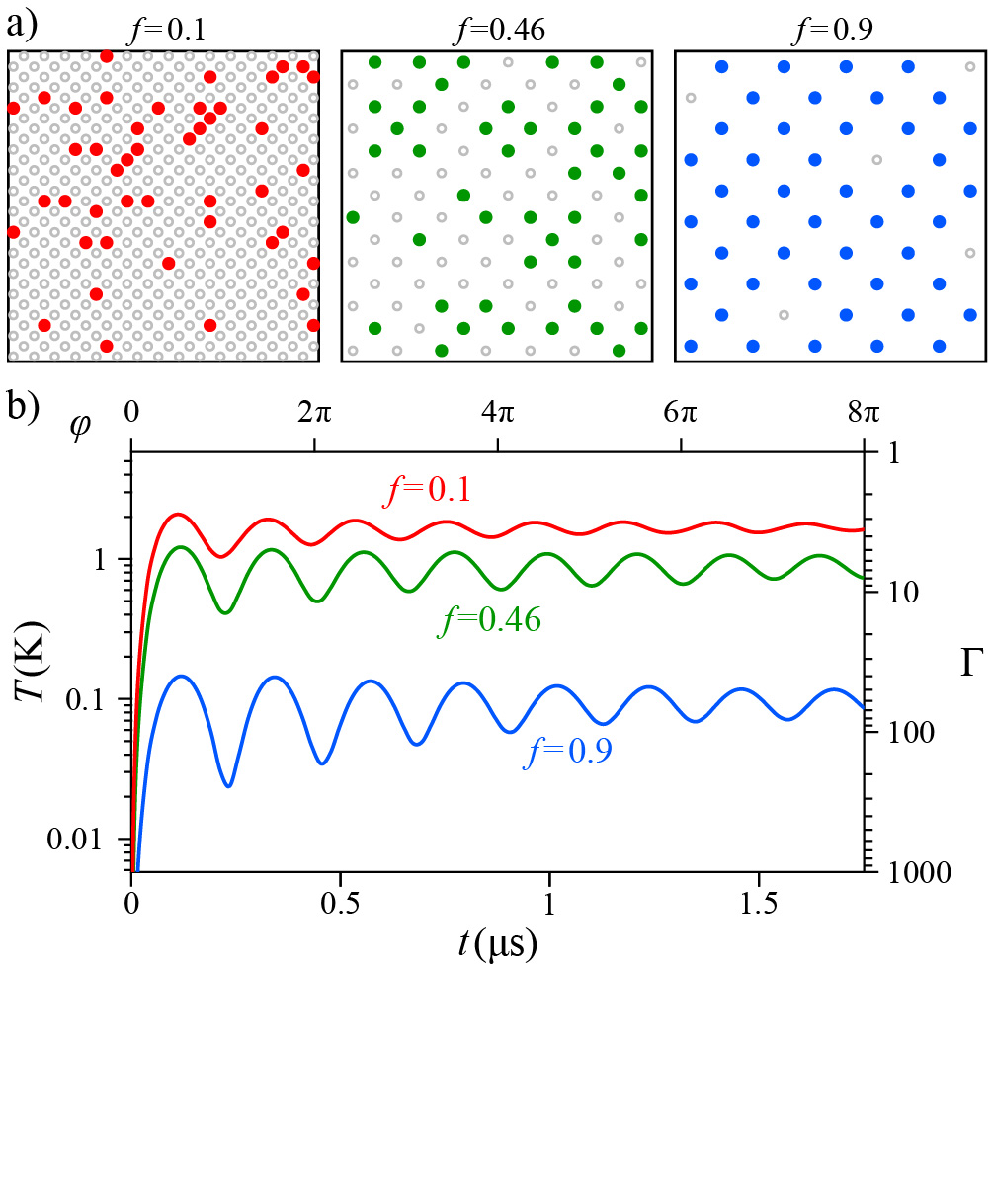
a) Illustration of lattice filling fractions ranging from small (f=0.1) to high (f=0.9). b) The temperature of ions following ionisation, with the increase due to disorder-induced heating. Higher filling fractions (and therefore more ordered systems) correspond to lower final temperatures.
New electron sources using laser-cooled atoms promise breakthrough enhancements in brightness with potential for ultrafast imaging at atomic scales. In these sources the beam current is intrinsically tied to the efficiency of excitation of the cold atoms. Here we present experiments where we use the counter-intuitive STIRAP technique to increase the excitation efficiency and therefore electron current by 60% compared to conventional incoherent methods.
Reference: B. M. Sparkes, D. Murphy, R. J. Taylor, R. W. Speirs, A. J. McCulloch, R. E. Scholten, Physical Review A 94, 023404 (2016) [pdf]
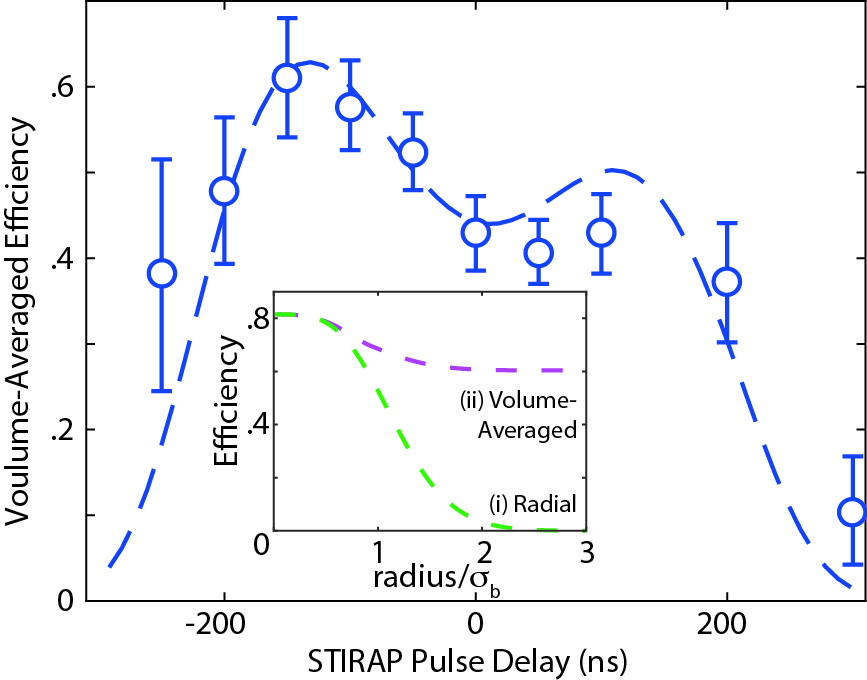
The volume-averaged efficiency as a function of the delay between the two STIRAP laser pulses (negative delay indicates the characteristic “counter-intuitive” pulse sequence). The inset shows the calculated efficiency as a function of integration radius, indicating a peak efficiency of 82%.
This invited article reviews cold atom electron sources, describing the many different implementations of the concept and an overview of the relevant theory behind their operation. We compare results from recent cold electron diffraction experiments with typical results achieved using conventional thermal sources, highlighting the potential of cold atom sources for single-shot and ultrafast diffraction images of non-crystalline objects.
Reference: A. J. McCulloch, B. M. Sparkes, R. E. Scholten, Journal of Physics B 49, 164004 (2016) [pdf]
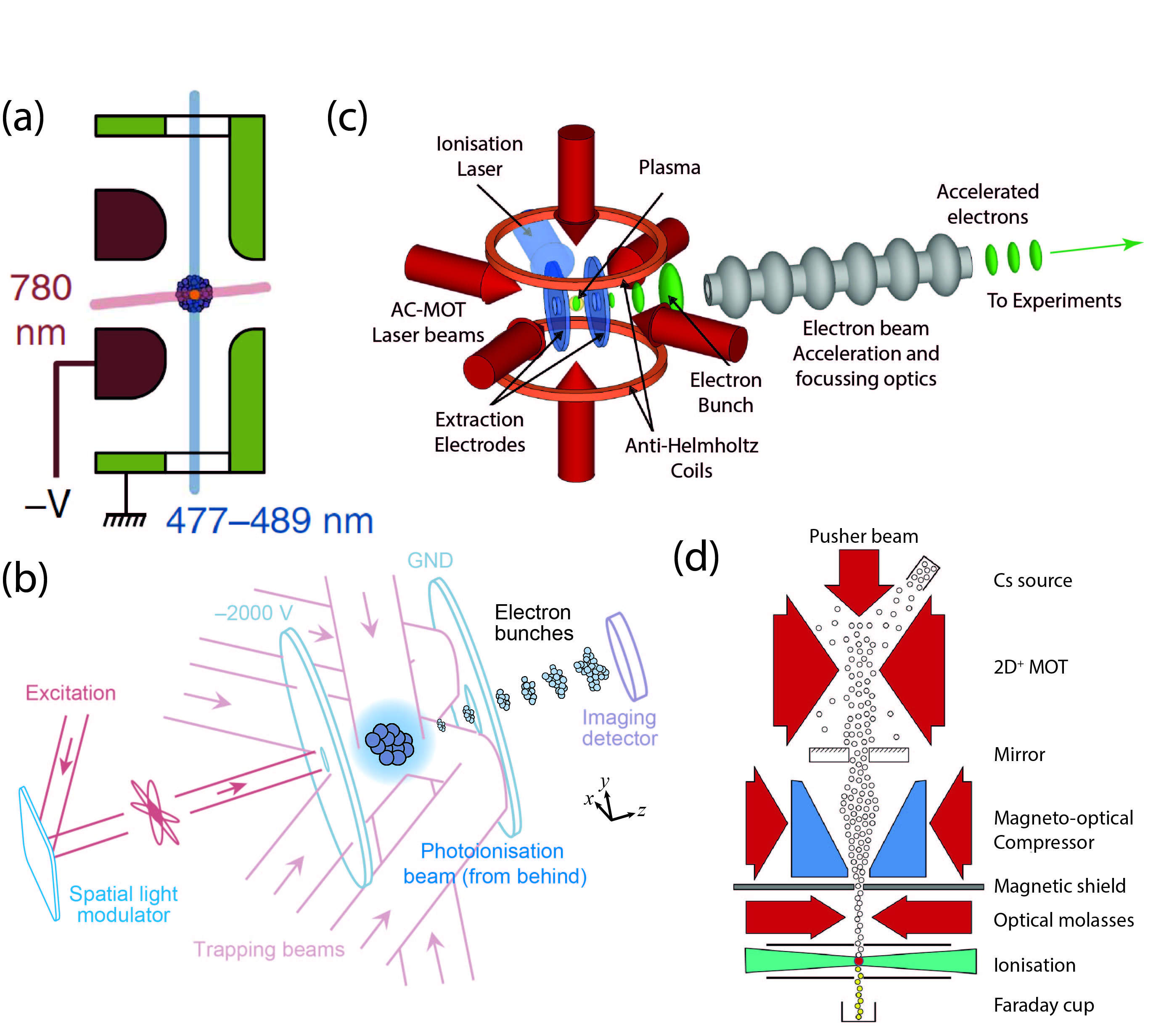
Stabilisation of laser frequency is essential for numerous applications, including atomic clocks, laser cooling and trapping of atoms, and Bose-Einstein condensation. We have shown that polarisation spectroscopy can achieve frequency narrowing to below 1kHz. The method is inherently tied to an absolute atomic resonance, and relatively simple compared to the more common approach of locking to an ultrastable optical cavity.
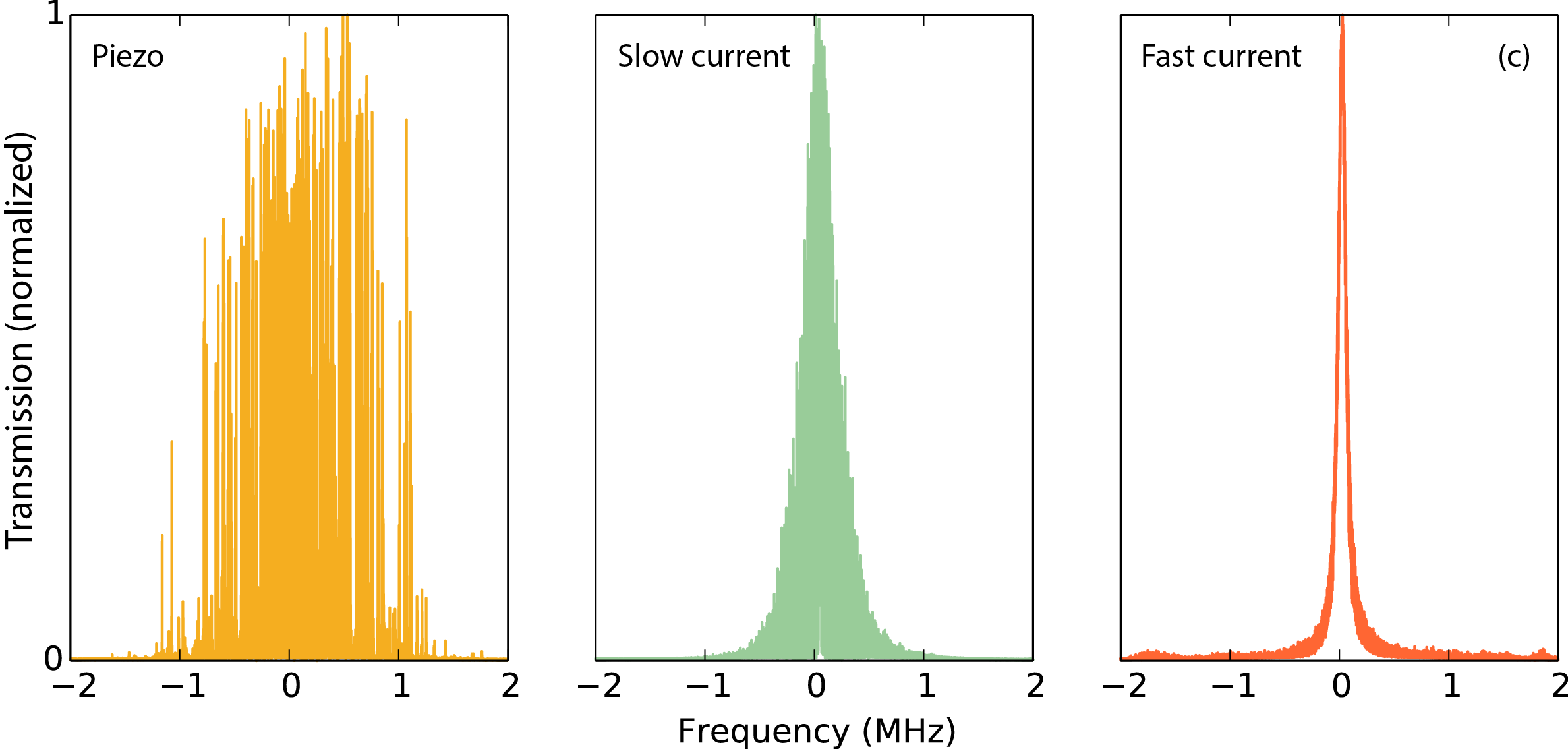
Laser transmission through an ultrastable optical cavity resonance. Frequency stabilisation feedback is via piezo only, and to current with slow and high bandwidth.
Reference: J. S. Torrance, B. M. Sparkes, L. D. Turner, R. E. Scholten, Optics Express 24, 11396 (2016) [pdf]
Our article Single-shot electron diffraction using a cold electron source, has been selected by the Editors of Journal of Physics B:Atomic, Molecular and Optical Physics for inclusion in their 'Highlights of 2015' collection due to "its outstanding quality and impact within the field of AMO physics".
Reference: R. W. Speirs, C. T. Putkunz, A. J. McCulloch, K. A. Nugent, B. M. Sparkes, R. E. Scholten, Journal of Physics B 48, 214002 (2015) [pdf]
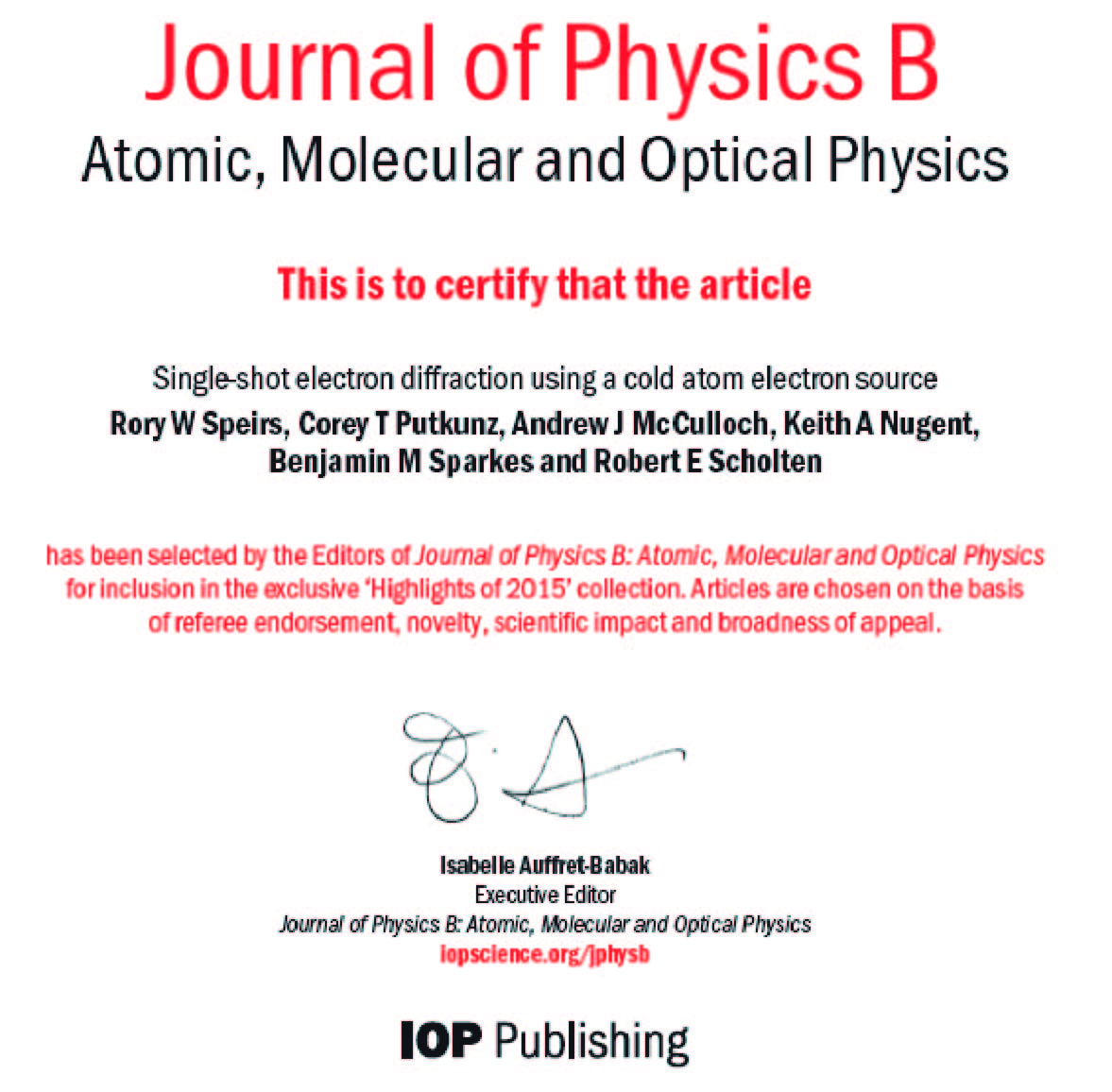
We have shown that the phenomena of Rydberg blockade can be used to increase the ordering of ions in a cold expanding bunch, leading to an increase in focusability for our cold atom ion source.
Reference: D. Murphy, R. E. Scholten, B. M. Sparkes, Physical Review Letters 115, 214802 (2015) [pdf]