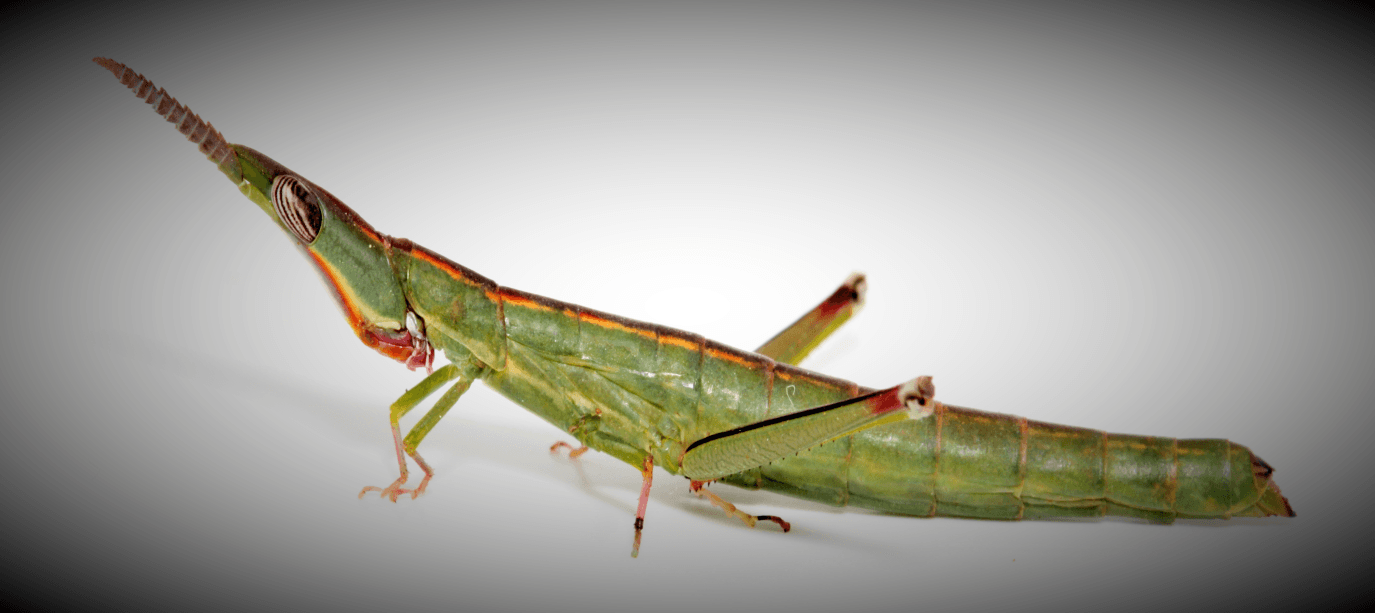
The diversity of Aussie grasshoppers | Part two
Words and images: Vanessa White
Some important lessons learnt and new questions around Vandiemenella laboratory rearing
In the previous grasshopper blog, I reported “reasonable success with room for improvement” in our attempts to rear Vandiemenella grasshopper nymphs in the laboratory. Alternative housing is an important focus for improvement, but a discussion with Mike and Ary raised the question of differing food requirements for adults and nymphs.
Studies have shown that some grasshopper species show changes in diet preference across their life cycle from hatchling to adult. Different grasshopper species may switch diets according to their life stage (Unsicker et al. 2008) or by sex (Asshoff and Hattenschwiler 2005). Feeding behaviour in the field is also complex. When the available plants in the environment are nutritionally poor, nymphs might practice polyphagy to overcome the nutrient deficit of any one food plant (Miura and Ohsaki 2004). A grasshopper might be able to develop normally with only one plant food, but a habitat with a mixture of foods is preferable (Ahmad and Nabi 2012).
Much like humans, grasshoppers have an array of microorganisms in their digestive tracts. This microbiota, composed of bacteria and fungi (including moulds) may play a role in assisting digestion and providing protection against toxins in plant food (Ademolu and Idowu 2011; Engel and Moran 2013). Rather than the microbiota being passed from mother to offspring, it seems that they acquire it from food after hatching (Ademolu and Idowu 2011; Engel and Moran 2013). Artificial diets and laboratory rearing might significantly alter grasshopper diets and, in turn, their gut microbiota (Jehmlich et al. 2016).
The “robust and Houdini-like” Anglesea population: One of my favourite Vandiemenella chromosome races to work with is viatica 19 collected from Anglesea. These were the first grasshoppers I ever caught in the field (with expert advice on technique from Ary), and they have proven robust under laboratory conditions and tolerate high temperature stress. They’re also very fertile. The Anglesea individuals have however provided a few headaches along the way. Their ability to escape has taught me a lot about Vandiemenella in general; they hide on dark clothing (often my dark clothing) and they use floor shadows and chair legs to avoid recapture. In March 2018, Ary returned from Anglesea with a sample of 5 males and 9 females. Mike and Ary have since revisited that particular site a few times to better understand the grasshopper’s life cycle. Information across time scales, including changes in abundance and in the proportions of the different life stages, is important for our understanding of local adaptation, and potentially the evolution of different reproductive strategies such as suspended development (diapause) which helps the grasshoppers synchronise nymph emergence with ideal conditions.
The majority of individuals from the first collection were 3rd instar nymphs. All 14 individuals were housed in a single rectangular cage (H = 45 cm * W = 30 cm * D = 35 cm). Three sides of the cage are mesh for ventilation and provides structure for the nymphs to climb. The mesh must also be very fine to prevent the tiny nymphs from escaping. The other sides are made of clear Perspex, the lower half of one side opens fully. This doorway also has a sliding window built into it, just large enough to fit a hand through to feed and clean the colony while minimising escapes. A circular tub of sand is recessed into the floor of the cage which is used for egg-laying. In the field females lay their eggs in sand and are known to bury themselves up to the head to ensure that eggs are laid deep enough (Mike Kearney, personal communication). The tub of sand allows caged females to lay their eggs at ground level, as they would in the field. Two additional tubs of sand which sit above ground level are provided as alternatives.
Food, water, temperature, and light conditions for the Anglesea population are identical to those of the adults housed in the “cup complex” (see part 1) except: (a) when feeding the nymphs, the mesh sides of the cage and the sand are sprayed with water, and (b) a small halogen light is placed directly over the cage for 8 hours a day to provide additional warmth. This additional warmth may be necessary for egg hatching, drying out the wet sand (periodic wetting and drying mimics field conditions), and to control humidity in the cage. High humidity might make moulting difficult for nymphs, making their skins sticky and difficult to break out of, as well as increasing risk of fungal infections.
Mike Kearney’s “tried and tested” grasshopper cage design: Spacious and well ventilated with mesh on the sides for climbing and a pot of sand built into the bottom of the cage (bottom left) where adult females can lay eggs. This type of cage is used to house the Vandiemenella Anglesea adults (the cage where the nymphs are born) and to run the nymph microbiota feeding experiment (“diverse” and “control” cages).
Babies, babies and more babies: I first noticed females laying eggs in the sand in June 2018, 3 months after the nymphs were collected. I have seen multiple females laying eggs in the tub of sand at the base of the cage, but none used the alternative tubs higher up in the cage. In the field, Vandiemenella is thought to be an annual species, where the adults die in summer after egg laying, with the eggs hatching in the following autumn. However Mike and Ary have found some live adults in summer, suggesting the story is more complicated. On 24 August (roughly two months after I noticed the first egg-laying) I was excited to observe freshly hatched first instar nymphs in the cage. Nymphs continued to emerge every day for a month and the cage housed 167 nymphs by 24 September. Total development time for the eggs was 57 days, a little longer than previously thought (Kawakami 2008). This may be because we maintained the cages at a lower temperature. Temperature has an immediate effect on the development time of insect eggs.
Despite regularly observing the cage I have never actually seen a nymph hatch during the day. So, I suspect the majority are hatching at night. This would be a useful strategy in the field to avoid diurnal predators. In the field, adults may cannibalise some nymphs, though I have never seen this in the lab. After hatching nymphs are usually found high up on the bidgee widgee foliage. The nymph’s mouthparts are extremely small, so it’s difficult to see, but I believe that they immediately begin feeding on emergence. So far I have found only one dead nymph in the cage, which is an excellent result compared to the Vandiemenella nymphs that were reared in petri dishes and vials which often didn’t moult properly and died trapped in their unshed skin. The spaciousness of the cage and lack of intervention in egg-laying probably has something to do with this (and also my fine insect-husbandry efforts), but the robustness of this particular population is probably also involved.
The healthy F1 (first generation) nymphs are now going to be used in experiments on feeding preference and development. Namely, do the nymphs and adults have different food preferences, and do the hatchlings benefit from a diverse diet rich in microbiota?
A tiny newly hatched Vandiemenella viatica nymph, coin for scale.
To test the effect of diet mixing on nymph development we will be running an experiment as follows: Two cages will each house 30 freshly hatched nymphs. In the “diverse” feeding cage nymphs are given a variety of foods (see below). The other is a control cage where nymphs are given a standard uniform diet. This is the same diet given to previous adult laboratory populations and the previous nymph trials with petri dishes and vials. The control provides a comparison to check the results of the diverse cage while holding all other variables constant. The diverse diet will have (depending on the availability) at least seven of these foods:
- Chrysocephalum apiculatum ramosissimum – common everlasting/yellow buttons (flowers and foliage)
- Chrysocephalum semipapposum (flowers and foliage)
- Acaena novaezelandiae – “bidgee widgee” (foliage)
- Spinifex grass roots
- Grass and clover
- Lichen and moss
- Orthoptera mix
- Bran flakes
- Frass (insect poo) collected from adults – this might play a role in the transfer of gut microbiota from one generation to another
- A pot of moist sandy soil – this may be an environmental source of beneficial microbes; the nymphs could walk over a surface rich in microbiota then clean their legs afterwards and thus acquire gut microbiota
The food will be placed at various heights, to give all nymphs equal access and to make sure that results aren’t confounded by nymphs that may be slightly better at accessing food. The control cage will have have bidgee widgee foliage and orthopteran mix, the standard diet for the adults.
The inside of the nymph microbiota feeding experiment “diverse” cage where Chrsyocephalum species (flowers and foliage) and other potentially “microbiota-rich” foods such as moss, lichen, roots of spinifex grass and adult frass (poo) are available.
The variables compared between the “diverse” and “control” cages) are: (1) the time taken for all nymphs to reach adulthood, (2) the final number of nymphs that reach adulthood, and (3) a summary of the nymphs’ presence on the different food sources in the “diverse” cage observed over the duration of the experiment.
Stay tuned for an update on the outcome of the microbiota feeding trial. I hope I’ve stimulated some interest in our Aussie grasshoppers, and an overview of the scientific process of data collection and experimentation. Spare a thought for our native fauna and what they have to offer us in terms of our understanding of evolution, local adaptation, conservation, and their ongoing contribution to a rich and unique Australian entomological history.