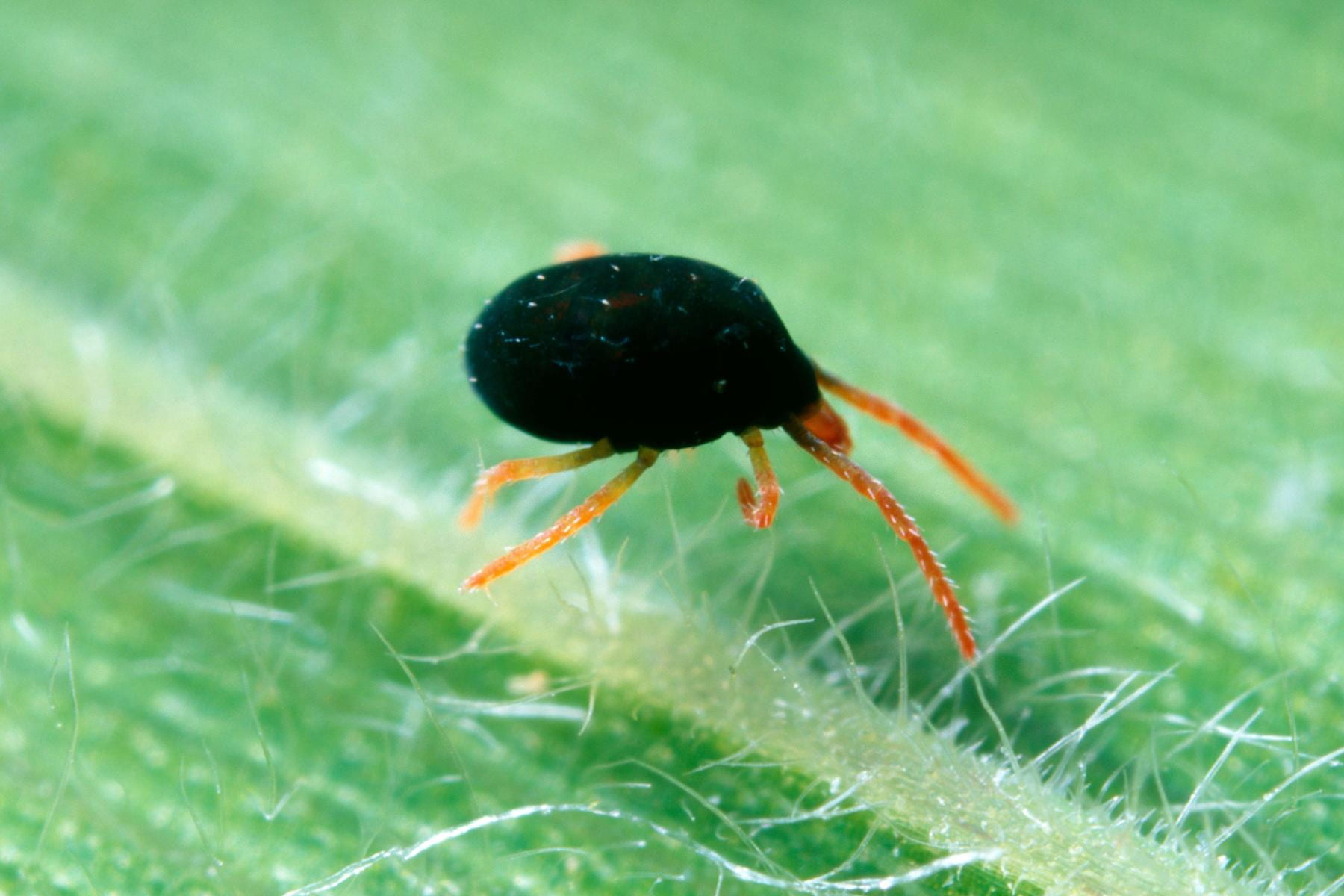
New Pursuit article – Have resistance, will travel
This article was first published on Pursuit. Read the original article here.
Authors: Dr Nancy Endersby-Harshman, Dr Qiong Yang, Dr Tom Schmidt and Professor Ary Hoffmann
Around the world, pest insects – like mosquitoes – often become resistant to the insecticides meant to control them, causing problems for agriculture and public health.
Resistance in multiple populations of a species might be due to independent evolution as a result of local spraying by farmers or the pattern of spray application in a local district.
But if resistance evolves in one population and those individuals or their descendants move elsewhere, they can spread their resistance to new populations – something called a ‘genetic invasion’.
The University of Melbourne’s Pest and Environmental Adaptation Research Group (PEARG), based at the Bio21 Institute, is shedding new light on how this resistance arises, investigating global geographical patterns of insecticide resistance and the evolutionary forces that produce them.
TRACKING GENETIC INVASIONS
The PEARG investigated resistance in Aedes aegypti, the main mosquito involved in dengue transmission in the Indo-Pacific region.
Mutations in the sodium channel gene of this mosquito are known to play a role in resistance to pyrethroid insecticides – one of the most common chemical groups used in mosquito control.
The sodium channel proteins found in insect cell membranes are essential for transmission of nerve impulses, but can be disrupted by the binding of pyrethroid insecticides, leading to death of the insect. Mutations in the sodium channel gene change the protein so that the insecticides cannot bind, allowing the pest insect to survive.
Our initial findings found that two types of pyrethroid resistance were common in Indo-Pacific Aedes aegypti, and each type of resistance was determined by a specific set of resistance mutations.
These two ‘resistance profiles’ are widely, but not uniformly, distributed across Aedes aegypti populations in the Indo-Pacific.
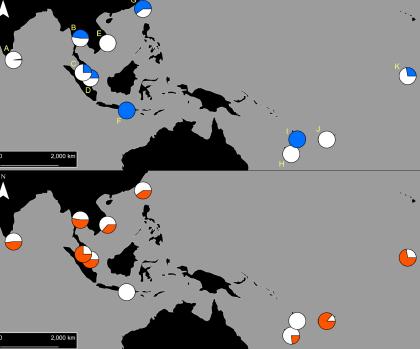
Our team then investigated patterns of movement of the two resistance profiles.
When a genetic invasion occurs, the mutations that create resistance are spread by migrating individual mosquitoes moving to a new population.
Looking only at these resistance mutations makes it impossible to tell whether a genetic invasion has occurred because identical resistance mutations that are highly beneficial to the mosquito could become common at multiple locations.
After all, the same insecticides are often used to control the same pests in different locations, and those insecticides will exert the same selection pressures on each mosquito population.
However, genetic invasions also transfer other genetic material into the new population.
This genetic material may confer no advantage to the organism. It is transferred simply because it occupies a position on the DNA strand within, or close, to the sodium channel gene.
So, it is ‘linked’ to the resistance mutations by proximity.
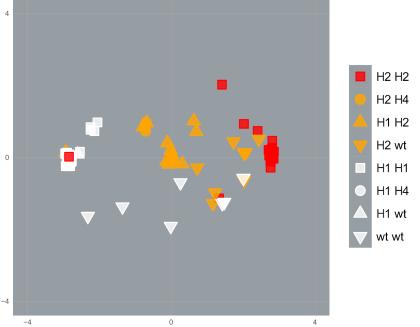
Using cutting-edge molecular analytical techniques, PEARG found that DNA near resistance mutations was similar among mosquitoes with the same resistance profile, even when they were from different populations that were genetically very distinct.
This indicates that a series of undocumented ‘genetic invasions’ have occurred in the Indo-Pacific region, as mosquitoes with resistance mutations have moved around.
These results highlight the importance of biosecurity controls to prevent resistance moving between populations. Currently, biosecurity focuses on species, but it is important to focus on incursions of harmful genes within species, as well as harmful species.
MANAGING EMERGING RESISTANCE
Our work on the redlegged earth mite, Halotydeus destructor, a major agricultural crop pest, tells a different story about pesticide resistance and movement.
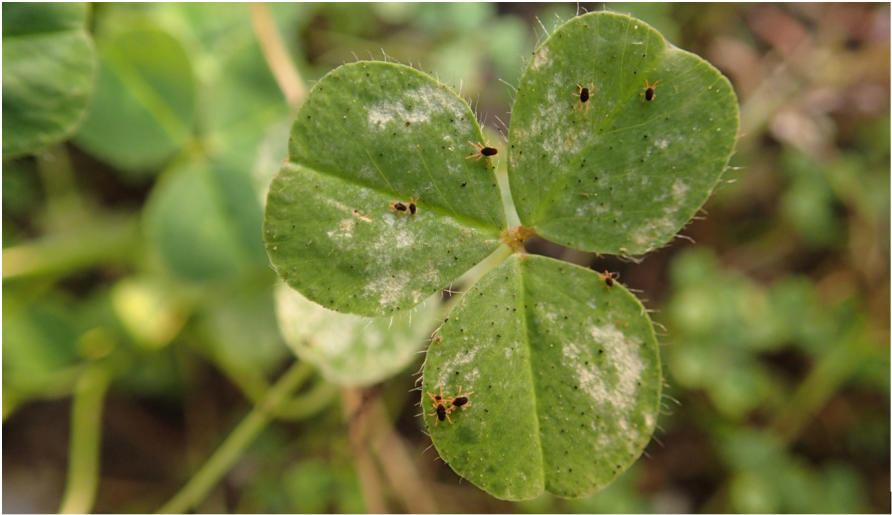
This invasive mite has been chemically controlled for over 50 years, but resistance to pyrethroid and organophosphate pesticides is developing. Most work on resistance has been limited to describing where resistance is found, but not understanding how it got there.
Are resistant mites being carried long distances across the country by wind currents, soil on machinery or in fodder? Or is resistance developing locally through individual evolutionary episodes?
Our new research explains processes behind emerging resistance, which is crucial for its management.
If resistance arises locally at one location, a high level of similarity in DNA can be expected between resistant samples from that location and adjacent susceptible samples as a result of ongoing gene flow and a common origin of the mites.
If resistant mites move to other areas and flourish, higher genomic differentiation between the resistant samples and nearby susceptible mites is expected.
FINDING THE LOCAL ORIGIN
Using the latest DNA technology, our team explored the local origin of pyrethroid resistance at a highly localised scale around Boyup Brook, in Western Australia.
Two sodium channel mutations associated with resistance were discovered there in 2013.
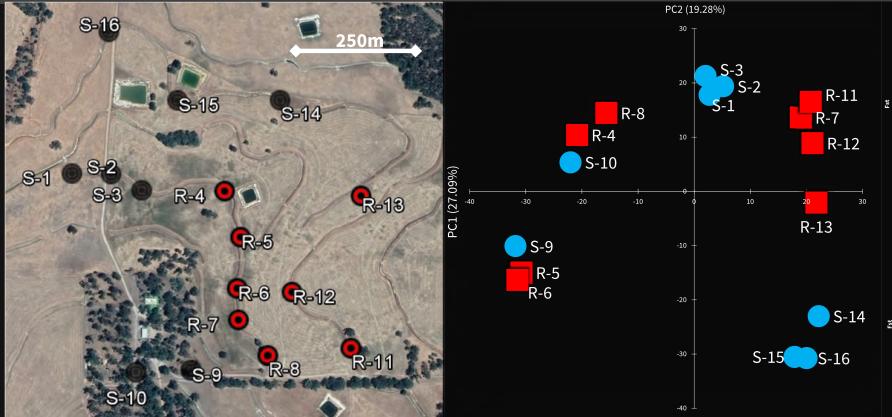
Genetic analysis showed nearby samples had similar DNA, particularly when they came from a location along the same fence line, suggesting mites tend to move along fences more easily than across paddocks.
At the regional level, genomic data showed there is close similarity in DNA between susceptible and resistant mites from the same locations where resistance has arisen in Western Australia and South Australia.
In contrast, the resistant mites in one part of the country are genomically different from those in other areas. This pattern indicates that, unlike in the mosquitoes, resistance outbreaks are local and aren’t spreading across regional or state boundaries.
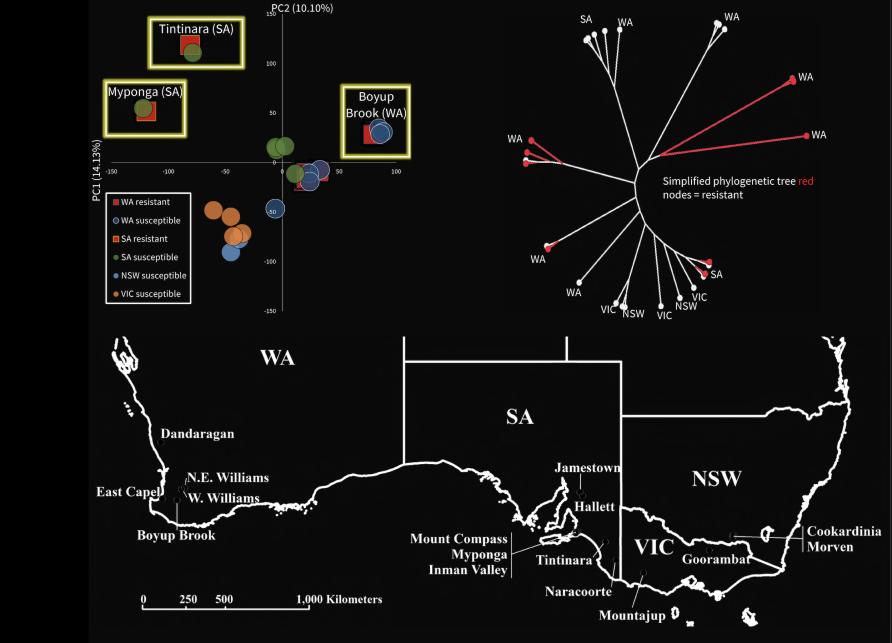
Our findings highlight that multiple independent evolutionary events lead to resistance in redlegged earth mites. This makes it critical to use the correct pesticide and crop management on each farm.
Reservoirs of susceptible mites should be maintained on properties, like along a fence line where pesticide applications could be avoided to prevent selection and local evolution.
This ensures that resistance doesn’t easily build up within farms.
Rotations of pesticides and non-chemical control strategies that reduce selection pressures are also important in decreasing any further local resistance evolution. This is advocated in the current National Resistance Management Strategy, supported by the Grains Research and Development Corporation.
Our research shows that pesticide resistance can arise in populations through very different means and, as a result, there are very different implications for resistance management.